- University of Kent
- School of Biosciences
- People
- Dr Christopher Mulligan
Dr Christopher Mulligan
Projects Lead for Undergraduate Programmes
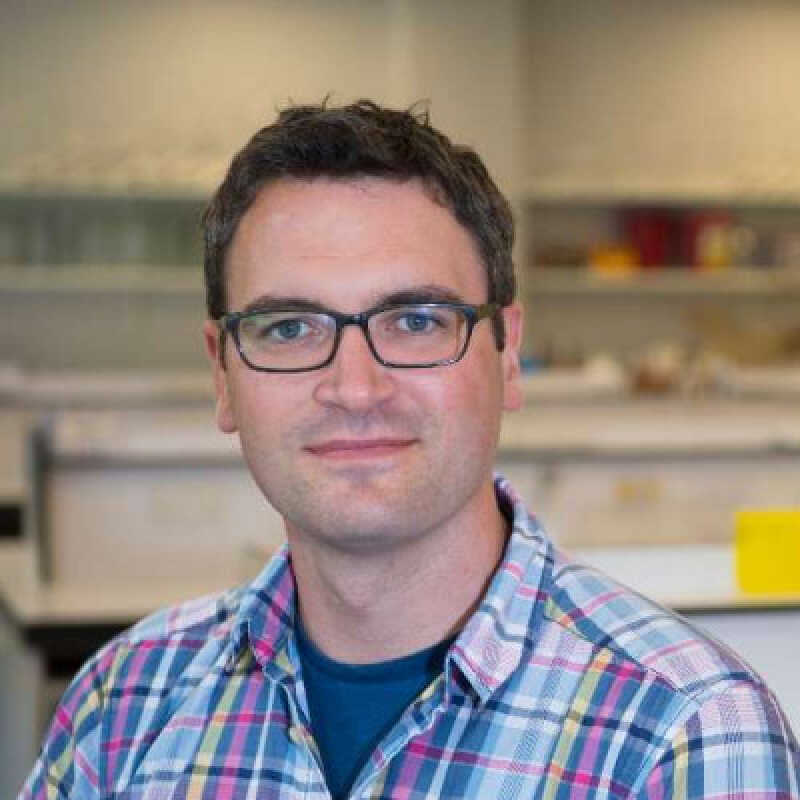
Dr Christopher Mulligan joined the School of Biosciences in May 2017. He obtained an undergraduate degree in Biochemistry from the University of York (2004), and then performed his thesis research with Dr Gavin Thomas at the University of York (2004-2008).
Subsequently, he took up a postdoctoral position in the same lab (2008-2011), where he studied the structure and function of transporters involved in the uptake of virulence factors into human pathogens.
Following his time in York, Chris obtained a visiting fellow position in Dr Joseph Mindell’s lab in the Membrane Transport Biophysics Section of the National Institute of Neurological Disorders and Stroke (NINDS) at the National Institutes of Health (NIH) in Maryland, USA. During his time at NIH (2011-2017), Chris studied the structure and mechanism of transport protein families involved in a number of key cellular functions, including neurotransmission and metabolic regulation.
Transport proteins play a major role in crucial cellular processes in all forms of life; from the uptake of nutrients and virulence factors to the extrusion of toxins (including antibiotics) in bacteria, as well as the regulation of neurotransmission and metabolism in mammals.
In humans, improper regulation and mutations in transporters are often associated with disease states like ALS, Alzheimer’s disease and epilepsy. Whereas, in bacteria, transporters contribute substantially to the virulence of human pathogens and their resistance to antibiotics, making them prime targets for new therapeutics. In addition, transporters are also key players in industrial biotechnology. Bacterial cell factories that are used to produce high value chemicals for industrial and medical purposes rely heavily on transport proteins to take up precursors and extrude the final products.
Transport proteins are highly dynamic molecular machines, most of which actively pump their substrates across the membrane fueled by an energy source; usually either ATP hydrolysis or electrochemical gradients (H+ and Na+ gradients). My lab uses a combination of biochemical, biophysical and microbiological techniques to understand the molecular mechanisms of transport proteins; how they recognise compounds, how they harness an energy source to pump compounds across the membrane, and how they move during transport. The more we understand about the mechanism of transporters, the better placed we will be to manipulate their function; either inhibiting them if they have undesirable effects, for example, antimicrobial resistance; or harnessing and enhancing their capabilities if they are useful, for example, for applications in industrial biotechnology.
Loading publications...
Showing of total publications in the Kent Academic Repository. View all publications